Here I'll be talking about my recently published paper. If you want a copy of any of the articles I talk about, contact me and I will share the PDFs I have the rights to share.
For a long while, I've been measuring the colours of Kuiper Belt Objects. That is, point a telescope at a target and take a picture, then switch to a different filter, and take another picture. The difference in brightness between the two filters provides a measure of the target's colour. The purpose of this is to get a handle on the composition of the source, and maybe where it came from. In much the same way that colour can tell if the beer you're about to drink is an ale or a stout, colour of a Kuiper Belt Object can tell us what kind of object we are looking at.
Compositionally speaking, there are two types of Kuiper Belt Object - the Less Red, and the Very Red. Exciting names, right? There is a lot of info packed into those names though. The surfaces of just about all Kuiper Belt Objects are icy, and organic rich. It's the organic that causes the red colouring. Astronomers think that the difference between the two types of surface is the type of organic. My colleague, Mike Brown, and I talked about this in detail in a paper we published back in 2012. More recently, Ian Wong and Mike together hypothesized that H2S is the culprit. That is, the Less Red objects formed closer to the Sun and essentially had their primordial content of sulphur (in the form of H2S) baked off, while the Very Red objects formed further away from the Sun and kept much of their sulphur. A few billion years of irradiation from Solar particles drove different chemistries on each surface, and voila! We have different surface types.
The above idea points to the idea that surface type can be used as a proxy for formation location. This is true regardless of whether the cause was sulphur or not. As long as objects gained their surface type due to formation distance from the Sun (the running hypothesis), then the surface types are excellent dynamical tracers in the sense that we can figure out how the gas giants moved, thereby moving objects from their primordial locations to where we currently find them. David Nesvorny published a great recent example of that type of work.
So this brings us to my most recent paper, which focuses on the colours of the Cold Classical Kuiper Belt Objects. These objects are named because of the dynamically cold - circular orbits, and constrained to the ecliptic - orbits. Original predictions of what the Kuiper Belt would look like said these are the types of orbits we'll see, and they were kind of right! You can see just how constrained to the ecliptic these bodies are in the animation below.
Much of what we know about where the Very Red objects formed is from the cold classicals. Almost all of them are very red. Some of the reddest objects in the Solar System are found in the cold classical region. What's more, we know that most formed right where we currently find them. We can say that with confidence, because many of them are actually binary bodies, with two (or more) objects orbiting each other, and that pair together in orbit around the Sun. Some of these binaries are so widely separated that we can even resolve them from the Earth. But it also makes them dynamically fragile. Gravitationally tug them, just a bit too much, and the binary unbinds into two single objects. That means that many or most of these objects could not have been pushed around by Neptune, like most other Kuiper Belt Objects. A great paper by Alex Parker talks about this idea.
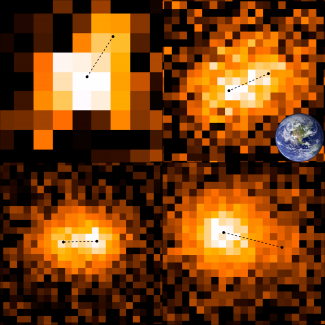
So it was weird then, in 2017, to find a rare type of binary object in the cold classical region, that has less red colours. We ended up calling these the blue binaries. It was pretty easy to show that these objects did not form alongside the very red cold classicals, and so we concluded that, at least the blue binaries are pushout contaminants. With some effort, I was able to show through simulation, that at some points during Neptune's outward migration, the conditions were just right to push objects out some, and land them in the cold classical region, all while preserving their binarity. Cool!
One surprising conclusion from our result though is the idea that those blue binaries all formed as binaries. That's just because there are no less red single bodies in the cold classical region. They formed as binary systems before they got pushed out. This was a wild idea, because it is formally incompatible with the normal way we thought about how planetesimals grow. Hierarchical accretion (objects of similar size crashing together and sticking to make bigger objects) doesn't form many binaries at all, let alone mainly binaries. Rather, this pointed to formation through gravitational collapse of a swarm of pebbles. This formation route preferentially forms binaries. You can read a bit more about this form of planetesimal growth in this post.
The discovery of the blue binaries drove some ideas that were a bit revolutionary, and like every revolutionary result, needs confirmation. This brings us to our newly published work. Since my 2017 paper, the Col-OSSOS team has substantially increased the number of colour measurements of cold classicals, including one more blue binary. The odd colour distribution of the binary cold classicals really stands out when compared to the single bodies.
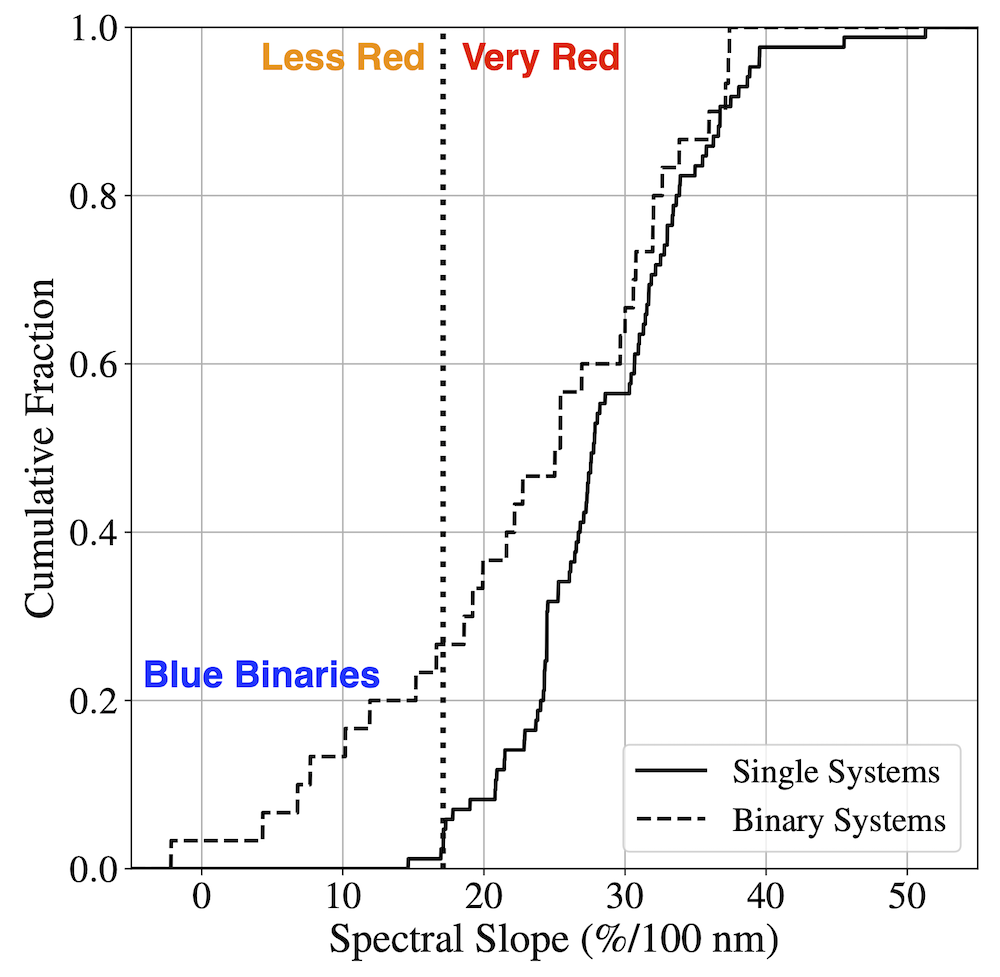
The increase in the number of colour measurements, and the discovery of another blue binary had confirmed all of our 2017 results. We are now in the position of starting to use these objects as specific tracers of dynamics and planet formation processes. Questions exist such as, how far did they get pushed out, or what conditions in the early Solar System favored formation through cloud collapse versus the "normal route" of hierarchical accretion? It's early days yet, and we have lots of work to do. Lots and lots of exciting work.